Cochlear synaptopathy
DOI:
https://doi.org/10.51445/sja.auditio.vol8.2024.103Keywords:
Hidden hearing loss, Audiology, Auditory nerve, Audiogram, Evoked potentials, Review article, Computational models, Physiology, synapseAbstract
Our understanding of cell structure damage in the peripheral auditory system due to acoustic overexposure and ageing underwent a paradigm shift with the discovery, over a decade ago, of cochlear synaptopathy (CS) – the permanent loss of synaptic connections between inner hair cells and auditory nerve fibres. Until then it was upheld that hair cells, and outer hair cells in particular, were the most vulnerable element in the peripheral auditory system. The classical paradigm of clinical audiological assessment has always been - and still is - based on measuring hearing thresholds with pure-tone audiometry. However, the discovery of CS has made it more urgent to develop new and more accurate diagnostic methods to detect hearing damage that is hidden in audiometry and to develop more specific tests for different types of peripheral cell damage. This article reviews the scientific literature on CS in animal models and discusses the evidence of CS in humans from cadaveric studies. Finally, after giving an overview of various inconclusive studies using psychoacoustic and physiological techniques in living humans, the article outlines some of the work currently underway in some European universities and future prospects for diagnosing and treating peripheral hearing loss.
Downloads
Visibility and Altmetrics
Metrics
References
Auerbach, B. D., Rodrigues, P. V., & Salvi, R. J. (2014). Central gain control in tinnitus and hyperacusis. Frontiers in Neurology, 5, 1-21, https://doi.org/10.3389/fneur.2014.00206.
Bharadwaj, H. M., Hustedt-Mai, A. R., Ginsberg, H. M., Dougherty, K. M., Muthaiah, V. P. K., Hagedorn, A., Simpson, J. M., & Heinz, M. G. (2022). Cross-species experiments reveal widespread cochlear neural damage in normal hearing. Communications Biology, 5, 733, https://doi.org/10.1038/s42003-022-03691-4.
Bharadwaj, H. M., Masud, S., Mehraei, G., Verhulst, S., & Shinn-Cunningham, B. G. (2015). Individual differences reveal correlates of hidden hearing deficits. The Journal of Neuroscience, 35, 2161-2172, https://doi.org/10.1523/JNEUROSCI.3915-14.2015.
Bramhall, N., Beach, E. F., Epp, B., Le Prell, C. G., Lopez-Poveda, E. A., Plack, C. J., Schaette, R., Verhulst, S., & Canlon, B. (2019). The search for noise-induced cochlear synaptopathy in humans: Mission impossible? Hearing Research, 377, 88-103, https://doi.org/10.1016/j.heares.2019.02.016.
Bramhall, N. F., Konrad-Martin, D., & McMillan, G. P. (2018a). Tinnitus and auditory perception after a history of noise exposure: Relationship to auditory brainstem response measures. Ear Hearing, 39, 881-894, https://doi.org/10.1097/AUD.0000000000000544.
Bramhall, N. F., McMillan, G. P., & Kampel, S. D. (2021). Envelope following response measurements in young veterans are consistent with noise-induced cochlear synaptopathy. Hearing Research, 408, 108310, https://doi.org/10.1016/j.heares.2021.108310.
Bramhall, N. F., McMillan, G. P., Kujawa, S. G., & Konrad-Martin, D. (2018b). Use of non-invasive measures to predict cochlear synapse counts. Hearing Research, 370, 113-119, https://doi.org/10.1016/j.heares.2018.10.006.
Bruce, I. C., Erfani, Y., & Zilany, M. S. (2018). A phenomenological model of the synapse between the inner hair cell and auditory nerve: Implications of limited neurotransmitter release sites. Hearing Research, 360, 40-54, https://doi.org/10.1016/j.heares.2017.12.016.
Buran, B. N., McMillan, G. P., Keshishzadeh, S., Verhulst, S., & Bramhall, N. F. (2022). Predicting synapse counts in living humans by combining computational models with auditory physiology. The Journal of the Acoustical Society of America, 151, 561-576, https://doi.org/10.1121/10.0009238.
Burkard, R. F. & Sims, D. (2001). The human auditory brainstem response to high click rates. American Journal of Audiology, 10, 53-61, https://doi.org/10.1044/1059-0889(2001/008).
Cantuaria, M. L., Pedersen, E. R., Waldorff, F. B., Sørensen, M., & Schmidt, J. H. (2021). Hearing examinations in southern denmark (hesd) database: a valuable tool for hearing-related epidemiological research. International Journal of Audiology, 60, 300-311, https://doi.org/10.1080/14992027.2020.1831702.
Caspary, D. M., Ling, L., Turner, J. G., & Hughes, L. F. (2008). Inhibitory neurotransmission, plasticity and aging in the mammalian central auditory system. Journal of Experimental Biology, 211, 1781-1791, https://doi.org/10.1242/jeb.013581.
Cassinotti, L. R., Ji, L., Borges, B. C., Cass, N. D., Desai, A. S., Kohrman, D. C., Liberman, M. C., & Corfas, G. (2022). Cochlear neurotrophin-3 overexpression at mid-life prevents age-related inner hair cell synaptopathy and slows age-related hearing loss. Aging Cell, 21, https://doi.org/10.1111/acel.13708.
Chambers, A. R., Resnik, J., Yuan, Y., Whitton, J. P., Edge, A. S., Liberman, M. C., & Polley, D. B. (2016). Central gain restores auditory processing following near-complete cochlear denervation. Neuron, 89, 867-879, https://doi.org/10.1016/j.neuron.2015.12.041.
Dau, T. (2003). The importance of cochlear processing for the formation of auditory brainstem and frequency following responses. The Journal of the Acoustical Society of America, 113, 936-950, https://doi.org/10.1121/1.1534833.
Diehl, P. U. & Schaette, R. (2015). Abnormal auditory gain in hyperacusis: Investigation with a computational model. Frontiers in Neurology, 6, 15, https://doi.org/10.3389/fneur.2015.00157.
Drakopoulos, F., Baby, D., & Verhulst, S. (2021). A convolutional neural-network framework for modelling auditory sensory cells and synapses. Communications Biology, 4, 827, https://doi.org/10.1038/s42003-021-02341-5.
Drakopoulos, F., Broucke, A. V. D., & Verhulst, S. (2023). A dnn-based hearing-aid strategy for real-time processing: One size fits all. IEEE https://ieeexplore.ieee.org/document/10094887/.
Drakopoulos, F., Vasilkov, V., Vecchi, A. O., Wartenberg, T., & Verhulst, S. (2022). Model-based hearing-enhancement strategies for cochlear synaptopathy pathologies. Hearing Research, 424, 108569, https://doi.org/10.1016/j.heares.2022.108569.
Drakopoulos, F. & Verhulst, S. (2023). A neural-network framework for the design of individualised hearing-loss compensation. IEEE/ACM Transactions on Audio, Speech, and Language Processing, 31, 2395-2409, https://doi.org/10.1109/TASLP.2023.3282093.
Eggermont, J. J. (2017). Effects of long-term non-traumatic noise exposure on the adult central auditory system. hearing problems without hearing loss. Hearing Research, 352, 12-22, https://doi.org/10.1016/j.heares.2016.10.015.
Encina-Llamas, G., Dau, T., & Epp, B. (2021). On the use of envelope following responses to estimate peripheral level compression in the auditory system. Scientific Reports, 11, 6962, https://doi.org/10.1038/s41598-021-85850-x.
Encina-Llamas, G., Harte, J. M., Dau, T., Shinn-Cunningham, B., & Epp, B. (2019). Investigating the effect of cochlear synaptopathy on envelope following responses using a model of the auditory nerve. Journal of the Association for Research in Otolaryngology, 20, 363-382, https://doi.org/10.1007/s10162-019-00721-7.
Fernandez, K. A., Jeffers, P. W., Lall, K., Liberman, M. C., & Kujawa, S. G. (2015). Aging after noise exposure: Acceleration of cochlear synaptopathy in “recovered” ears. The Journal of Neuroscience, 35, 7509-7520, https://doi.org/10.1523/JNEUROSCI.5138-14.2015.
Foster, A. C., Jacques, B. E., & Piu, F. (2022a). Hearing loss: The final frontier of pharmacology. Pharmacology Research Perspectives, 10, e00970, https://doi.org/10.1002/prp2.970.
Foster, A. C., Szobota, S., Piu, F., Jacques, B. E., Moore, D. R., Sanchez, V. A., & Anderson, J. J. (2022b). A neurotrophic approach to treating hearing loss: Translation from animal models to clinical proof-of-concept. The Journal of the Acoustical Society of America, 151, 3937-3946, https://doi.org/10.1121/10.0011510.
Fulbright, A., Prell, C. L., Griffiths, S., & Lobarinas, E. (2017). Effects of recreational noise on threshold and suprathreshold measures of auditory function. Seminars in Hearing, 38, 298-318, https://doi.org/10.1055/s-0037-1606325.
Furman, A. C., Kujawa, S. G., & Liberman, M. C. (2013). Noise-induced cochlear neuropathy is selective for fibers with low spontaneous rates. Journal of Neurophysiology, 110, 577-586, https://doi.org/10.1152/jn.00164.2013.
Grant, K. J., Mepani, A. M., Wu, P., Hancock, K. E., de Gruttola, V., Liberman, M. C., & Maison, S. F. (2020). Electrophysiological markers of cochlear function correlate with hearing-in-noise performance among audiometrically normal subjects. Journal of Neurophysiology, 124, 418-431, https://doi.org/10.1152/jn.00016.2020.
Grinn, S. K., Wiseman, K. B., Baker, J. A., & Prell, C. G. L. (2017). Hidden hearing loss? no effect of common recreational noise exposure on cochlear nerve response amplitude in humans. Frontiers in Neuroscience, 11, https://doi.org/10.3389/fnins.2017.00465.
Grose, J. H., Buss, E., & Elmore, H. (2019). Age-related changes in the auditory brainstem response and suprathreshold processing of temporal and spectral modulation. Trends in Hearing, 23, 233121651983961, https://doi.org/10.1177/2331216519839615.
Grose, J. H., Buss, E., & Hall, J. W. (2017). Loud music exposure and cochlear synaptopathy in young adults: Isolated auditory brainstem response effects but no perceptual consequences. Trends in Hearing, 21, 233121651773741, https://doi.org/10.1177/2331216517737417.
Guest, H., Munro, K. J., & Plack, C. J. (2017a). Tinnitus with a normal audiogram: Role of high-frequency sensitivity and reanalysis of brainstem-response measures to avoid audiometric over-matching. Hearing Research, 356, 116-117, https://doi.org/10.1016/j.heares.2017.10.002.
Guest, H., Munro, K. J., Prendergast, G., Howe, S., & Plack, C. J. (2017b). Tinnitus with a normal audiogram: Relation to noise exposure but no evidence for cochlear synaptopathy. Hearing Research, 344, 265-274, https://doi.org/10.1016/j.heares.2016.12.002.
Guest, H., Munro, K. J., Prendergast, G., Millman, R. E., & Plack, C. J. (2018). Impaired speech perception in noise with a normal audiogram: No evidence for cochlear synaptopathy and no relation to lifetime noise exposure. Hearing Research, 364, 142—151, https://doi.org/10.1016/j.heares.2018.03.008.
Guest, H., Munro, K. J., Prendergast, G., & Plack, C. J. (2019). Reliability and interrelations of seven proxy measures of cochlear synaptopathy. Hearing Research, 375, 34-43, https://doi.org/10.1016/j.heares.2019.01.018.
Hashimoto, K., Hickman, T. T., Suzuki, J., Ji, L., Kohrman, D. C., Corfas, G., & Liberman, M. C. (2019). Protection from noise-induced cochlear synaptopathy by virally mediated overexpression of nt3. Scientific Reports, 9, 15362, https://doi.org/10.1038/s41598-019-51724-6.
Heeringa, A. & van Dijk, P. (2014). The dissimilar time course of temporary threshold shifts and reduction of inhibition in the inferior colliculus following intense sound exposure. Hearing Research, 312, 38-47, https://doi.org/10.1016/j.heares.2014.03.004.
Hickman, T. T., Hashimoto, K., Liberman, L. D., & Liberman, M. C. (2020). Synaptic migration and reorganization after noise exposure suggests regeneration in a mature mammalian cochlea. Scientific Reports, 10, 19945, https://doi.org/10.1038/s41598-020-76553-w.
Hickman, T. T., Hashimoto, K., Liberman, L. D., & Liberman, M. C. (2021). Cochlear synaptic degeneration and regeneration after noise: Effects of age and neuronal subgroup. Frontiers in Cellular Neuroscience, 15, https://doi.org/10.3389/fncel.2021.684706.
Hickman, T. T., Smalt, C., Bobrow, J., Quatieri, T., & Liberman, M. C. (2018). Blast-induced cochlear synaptopathy in chinchillas. Scientific Reports, 8, 10740, https://doi.org/10.1038/s41598-018-28924-7.
Hickox, A. E., Larsen, E., Heinz, M. G., Shinobu, L., & Whitton, J. P. (2017). Translational issues in cochlear synaptopathy. Hearing Research, 349, 164-171, https://doi.org/10.1016/j.heares.2016.12.010.
Hind, S. E., Haines-Bazrafshan, R., Benton, C. L., Brassington, W., Towle, B., & Moore, D. R. (2011). Prevalence of clinical referrals having hearing thresholds within normal limits. International Journal of Audiology, 50, 708-716, https://doi.org/10.3109/14992027.2011.582049.
Johannesen, P. T., Buzo, B. C., & Lopez-Poveda, E. A. (2019). Evidence for age-related cochlear synaptopathy in humans unconnected to speech-in-noise intelligibility deficits. Hearing Research, 374, 35-48, https://doi.org/10.1016/j.heares.2019.01.017.
Johannesen, P. T., Leclere, T., Wijetillake, A., Segovia-Martínez, M., & Lopez-Poveda, E. A. (2022). Modeling temporal information encoding by the population of fibers in the healthy and synaptopathic auditory nerve. Hearing Research, 426, 108621, https://doi.org/10.1016/j.heares.2022.108621.
Johannesen, P. T. & Lopez-Poveda, E. A. (2021). Age-related central gain compensation for reduced auditory nerve output for people with normal audiograms, with and without tinnitus. iScience, 24, 102658, https://doi.org/10.1016/j.isci.2021.102658.
Keshishzadeh, S., Garrett, M., Vasilkov, V., & Verhulst, S. (2020). The derived-band envelope following response and its sensitivity to sensorineural hearing deficits. Hearing Research, 392, 107979, https://doi.org/10.1016/j.heares.2020.107979.
Keshishzadeh, S., Garrett, M., & Verhulst, S. (2021). Towards personalized auditory models: Predicting individual sensorineural hearing-loss profiles from recorded human auditory physiology. Trends in Hearing, 25, 233121652098840, https://doi.org/10.1177/2331216520988406.
Knipper, M., Dijk, P. V., Nunes, I., Rüttiger, L., & Zimmermann, U. (2013). Advances in the neurobiology of hearing disorders: Recent developments regarding the basis of tinnitus and hyperacusis. Progress in Neurobiology, 111, 17-33, https://doi.org/10.1016/j.pneurobio.2013.08.002.
Kujawa, S. G. & Liberman, M. C. (2009). Adding insult to injury: Cochlear nerve degeneration after “temporary” noise-induced hearing loss. The Journal of Neuroscience, 29, 14077-14085, https://doi.org/10.1523/JNEUROSCI.2845-09.2009.
Kumar, G., Amen, F., & Roy, D. (2007). Normal hearing tests: is a further appointment really necessary? Journal of the Royal Society of Medicine, 100, 66-66, https://doi.org/10.1258/jrsm.100.2.66-a.
Lai, J., Sommer, A. L., & Bartlett, E. L. (2017). Age-related changes in envelope-following responses at equalized peripheral or central activation. Neurobiology of Aging, 58, 191-200, https://doi.org/10.1016/j.neurobiolaging.2017.06.013.
Leake, P. A., Akil, O., & Lang, H. (2020). Neurotrophin gene therapy to promote survival of spiral ganglion neurons after deafness. Hearing Research, 394, 107955, https://doi.org/10.1016/j.heares.2020.107955.
Le Prell, C. G., Siburt, H. W., Lobarinas, E., Griffiths, S. K., & Spankovich, C. (2018). No reliable association between recreational noise exposure and threshold sensitivity, distortion product otoacoustic emission amplitude, or word-in-noise performance in a college student population. Ear Hearing, 39, 1057-1074, https://doi.org/10.1097/AUD.0000000000000575.
Liberman, M. & Kiang, N. Y.-S. (1984). Single-neuron labeling and chronic cochlear pathology. iv. stereocilia damage and alterations in rate- and phase-level functions. Hearing Research, 16, 75-90, https://doi.org/10.1016/0378-5955(84)90026-1.
Liberman, M. C. (1978). Auditory-nerve response from cats raised in a low-noise chamber. The Journal of the Acoustical Society of America, 63, 442-455, https://doi.org/10.1121/1.381736.
Liberman, M. C. (1982). Single-neuron labeling in the cat auditory nerve. Science, 216, 1239-1241, https://doi.org/10.1126/science.7079757.
Liberman, M. C., Epstein, M. J., Cleveland, S. S., Wang, H., & Maison, S. F. (2016). Toward a differential diagnosis of hidden hearing loss in humans. PLOS ONE, 11, e0162726, https://doi.org/10.1371/journal.pone.0162726.
Liberman, M. C. & Kujawa, S. G. (2017). Cochlear synaptopathy in acquired sensorineural hearing loss: Manifestations and mechanisms. Hearing Research, 349, 138-147, https://doi.org/10.1016/j.heares.2017.01. 003.
Lin, H. W., Furman, A. C., Kujawa, S. G., & Liberman, M. C. (2011). Primary neural degeneration in the guinea pig cochlea after reversible noise-induced threshold shift. Journal of the Association for Research in Otolaryngology, 12, 605-616, https://doi.org/10.1007/s10162-011-0277-0.
Liu, J., Stohl, J., & Overath, T. (2024). Hidden hearing loss: Fifteen years at a glance. Hearing Research, 443, 108967, https://doi.org/10.1016/j.heares.2024.108967.
Liu, L., Wang, H., Shi, L., Almuklass, A., He, T., Aiken, S., Bance, M., Yin, S., & Wang, J. (2012). Silent damage of noise on cochlear afferent innervation in guinea pigs and the impact on temporal processing. PLoS ONE, 7, e49550, https://doi.org/10.1371/journal.pone.0049550.
Lobarinas, E., Salvi, R., & Ding, D. (2013). Insensitivity of the audiogram to carboplatin induced inner hair cell loss in chinchillas. Hearing Research, 302, 113-120, https://doi.org/10.1016/j.heares.2013.03.012.
Lobarinas, E., Spankovich, C., & Le Prell, C. G. (2017). Evidence of “hidden hearing loss” following noise exposures that produce robust tts and abr wave-i amplitude reductions. Hearing Research, 349, 155-163, https://doi.org/10.1016/j.heares.2016.12.009.
Lopez-Poveda, E. A. (2014). Why do I hear but not understand? Stochastic undersampling as a model of degraded neural encoding of speech. Frontiers in Neuroscience, 8, 348, https://doi.org/10.3389/fnins.2014.00348.
Lopez-Poveda, E. A. & Barrios, P. (2013). Perception of stochastically undersampled sound waveforms: a model of auditory deafferentation. Frontiers in Neuroscience, 7, 124, https://doi.org/10.3389/fnins.2013.00124.
Maele, T. V., Keshishzadeh, S., Poortere, N. D., Dhooge, I., Keppler, H., & Verhulst, S. (2021). The variability in potential biomarkers for cochlear synaptopathy after recreational noise exposure. Journal of Speech, Language, and Hearing Research, 64, 4964-4981, https://doi.org/10.1044/2021_JSLHR-21-00064.
Makary, C. A., Shin, J., Kujawa, S. G., Liberman, M. C., & Merchant, S. N. (2011). Age-related primary cochlear neuronal degeneration in human temporal bones. Journal of the Association for Research in Otolaryngology, 12, 711-717, https://doi.org/10.1007/s10162-011-0283-2.
Märcher-Rørsted, J., Encina-Llamas, G., Dau, T., Liberman, M. C., Wu, P., & Hjortkjær, J. (2022). Age- related reduction in frequency-following responses as a potential marker of cochlear neural degeneration. Hearing Research, 414, 108411, https://doi.org/10.1016/j.heares.2021.108411.
Marmel, F., Rodríguez-Mendoza, M. A., & Lopez-Poveda, E. A. (2015). Stochastic undersampling steepens auditory threshold/duration functions: implications for understanding auditory deafferentation and aging. Frontiers in aging neuroscience, 7, 63, https://doi.org/10.3389/fnagi.2015.00063.
Maxwell, K. S., Robinson, J. M., Hoffmann, I., Hou, H. J., Searchfield, G., Baguley, D. M., McMurry, G., Piu, F., & Anderson, J. J. (2021). Intratympanic administration of oto-313 reduces tinnitus in patients with moderate to severe, persistent tinnitus: A phase 1/2 study. Otology Neurotology, 42, e1625-e1633, https://doi.org/10.1097/MAO.0000000000003369.
Mehraei, G., Hickox, A. E., Bharadwaj, H. M., Goldberg, H., Verhulst, S., Liberman, M. C., & Shinn-Cunningham, B. G. (2016). Auditory brainstem response latency in noise as a marker of cochlear synaptopathy. The Journal of Neuroscience, 36, 3755-3764, https://doi.org/10.1523/JNEUROSCI.4460-15.2016.
Mepani, A. M., Verhulst, S., Hancock, K. E., Garrett, M., Vasilkov, V., Bennett, K., de Gruttola, V., Liberman, M. C., & Maison, S. F. (2021). Envelope following responses predict speech-in-noise performance in normal- hearing listeners. Journal of Neurophysiology, 125, 1213-1222, https://doi.org/10.1152/jn.00620.2020.
Möhrle, D., Hofmeier, B., Amend, M., Wolpert, S., Ni, K., Bing, D., Klose, U., Pichler, B., Knipper, M., & Rüttiger, L. (2019). Enhanced central neural gain compensates acoustic trauma-induced cochlear impairment, but unlikely correlates with tinnitus and hyperacusis. Neuroscience, 407, 146-169, https://doi.org/10.1016/j.neuroscience.2018.12.038.
Möhrle, D., Ni, K., Varakina, K., Bing, D., Lee, S. C., Zimmermann, U., Knipper, M., & Rüttiger, L. (2016). Loss of auditory sensitivity from inner hair cell synaptopathy can be centrally compensated in the young but not old brain. Neurobiology of Aging, 44, 173-184, https://doi.org/10.1016/j.neurobiolaging.2016.05.001.
Parthasarathy, A., Bartlett, E. L., & Kujawa, S. G. (2019). Age-related changes in neural coding of envelope cues: Peripheral declines and central compensation. Neuroscience, 407, 21-31, https://doi.org/10.1016/j.neuroscience.2018.12.007.
Parthasarathy, A. & Kujawa, S. G. (2018). Synaptopathy in the aging cochlea: Characterizing early- neural deficits in auditory temporal envelope processing. The Journal of Neuroscience, 38, 7108-7119, https://doi.org/10.1523/JNEUROSCI.3240-17.2018.
Paul, B. T., Bruce, I. C., & Roberts, L. E. (2017). Evidence that hidden hearing loss underlies amplitude modulation encoding deficits in individuals with and without tinnitus. Hearing Research, 344, 170-182, https://doi.org/10.1016/j.heares.2016.11.010.
Peñuela, J. M., Oyarzabal, S. M., Batalla, F. N., López, R. H. S., & Herrero, T. V. (2022). Manual de audiología laboral. Lettera publicaciones. https://letterapublicaciones.com/producto/manual-de-audiologia-laboral/.
Prendergast, G., Guest, H., Munro, K. J., Kluk, K., Léger, A., Hall, D. A., Heinz, M. G., & Plack, C. J. (2017a). Effects of noise exposure on young adults with normal audiograms I: Electrophysiology. Hearing Research, 344, 68-81, https://doi.org/10.1016/j.heares.2016.10.028.
Prendergast, G., Millman, R. E., Guest, H., Munro, K. J., Kluk, K., Dewey, R. S., Hall, D. A., Heinz, M. G., & Plack, C. J. (2017b). Effects of noise exposure on young adults with normal audiograms II: Behavioral measures. Hearing Research, 356, 74-86, https://doi.org/10.1016/j.heares.2017.10.007.
Prendergast, G., Tu, W., Guest, H., Millman, R. E., Kluk, K., Couth, S., Munro, K. J., & Plack, C. J. (2018). Supra-threshold auditory brainstem response amplitudes in humans: Test-retest reliability, electrode montage and noise exposure. Hearing Research, 364, 38-47, https://doi.org/10.1016/j.heares.2018.04.002.
Ridley, C. L., Kopun, J. G., Neely, S. T., Gorga, M. P., & Rasetshwane, D. M. (2018). Using thresholds in noise to identify hidden hearing loss in humans. Ear Hearing, 39, 829-844, https://doi.org/10.1097/AUD.0000000000000543.
Roberts, L. E., Paul, B. T., & Bruce, I. C. (2018). Erratum and comment: Envelope following responses in normal hearing and in tinnitus. Hearing Research, 361, 157-158, https://doi.org/10.1016/j.heares.2018.01.011.
Rumschlag, J. A., McClaskey, C. M., Dias, J. W., Kerouac, L. B., Noble, K. v., Panganiban, C., Lang, H., & Harris, K. C. (2022). Age-related central gain with degraded neural synchrony in the auditory brainstem of mice and humans. Neurobiology of Aging, 115, 50-59, https://doi.org/10.1016/j.neurobiolaging.2022.03.014.
Ryan, A. & Dallos, P. (1975). Effect of absence of cochlear outer hair cells on behavioural auditory threshold. Nature, 253, 44-6, https://doi.org/10.1038/253044a0.
Salvi, R., Sun, W., Ding, D., Chen, G.-D., Lobarinas, E., Wang, J., Radziwon, K., & Auerbach, B. D. (2017). Inner hair cell loss disrupts hearing and cochlear function leading to sensory deprivation and enhanced central auditory gain. Frontiers in Neuroscience, 10, 1-14, https://doi.org/10.3389/fnins.2016.00621.
Saunders, G. H. & Haggard, M. P. (1989). The clinical assessment of obscure auditory dysfunction— 1. Auditory and psychological factors. Ear and Hearing, 10, 200-208, https://doi.org/10.1097/00003446-198906000-00011.
Schaette, R. (2014). Tinnitus in men, mice (as well as other rodents), and machines. Hearing Research, 311, 63-71, https://doi.org/10.1016/j.heares.2013.12.004.
Schaette, R. & McAlpine, D. (2011). Tinnitus with a normal audiogram: Physiological evidence for hidden hearing loss and computational model. The Journal of Neuroscience, 31, 13452-13457, https://doi.org/10.1523/JNEUROSCI.2156-11.2011.
Schuknecht, H. F. & Woellner, R. C. (1955). An experimental and clinical study of deafness from lesions of the cochlear nerve. The Journal of Laryngology Otology, 69, 75-97, https://doi.org/10.1017/S0022215100050465.
Sergeyenko, Y., Lall, K., Liberman, M. C., & Kujawa, S. G. (2013). Age-related cochlear synaptopathy: An early-onset contributor to auditory functional decline. The Journal of Neuroscience, 33, 13686-13694, https://doi.org/10.1523/JNEUROSCI.1783-13.2013.
Sheppard, A., Liu, X., Ding, D., & Salvi, R. (2018). Auditory central gain compensates for changes in cochlear output after prolonged low-level noise exposure. Neuroscience Letters, 687, 183-188, https://doi.org/10.1016/j.neulet.2018.09.054.
Shi, L., Liu, L., He, T., Guo, X., Yu, Z., Yin, S., & Wang, J. (2013). Ribbon synapse plasticity in the cochleae of guinea pigs after noise-induced silent damage. PLoS ONE, 8, e81566, https://doi.org/10.1371/journal.pone.0081566.
Skoe, E. & Tufts, J. (2018). Evidence of noise-induced subclinical hearing loss using auditory brainstem responses and objective measures of noise exposure in humans. Hearing Research, 361, 80-91, https://doi.org/10.1016/j.heares.2018.01.005.
Spankovich, C., Prell, C. G. L., Lobarinas, E., & Hood, L. J. (2017). Noise history and auditory function in young adults with and without type 1 diabetes mellitus. Ear Hearing, 38, 724-735, https://doi.org/10.1097/AUD.0000000000000457.
Stamper, G. C. & Johnson, T. A. (2015). Auditory function in normal-hearing, noise-exposed human ears. Ear Hearing, 36, 172-184, https://doi.org/10.1097/AUD.0000000000000107.
Suthakar, K. & Liberman, M. C. (2021). Auditory-nerve responses in mice with noise-induced cochlear synaptopathy. Journal of Neurophysiology, 126, 2027-2038, https://doi.org/10.1152/jn.00342.2021.
Suzuki, J., Corfas, G., & Liberman, M. C. (2016). Round-window delivery of neurotrophin 3 regenerates cochlear synapses after acoustic overexposure. Scientific Reports, 6, 24907, https://doi.org/10.1038/srep24907.
Taberner, A. M. & Liberman, M. C. (2005). Response properties of single auditory nerve fibers in the mouse. Journal of Neurophysiology, 93, 557-569, https://doi.org/10.1152/jn.00574.2004.
Temboury-Gutierrez, M., Encina-Llamas, G., & Dau, T. (2024a). Predicting early auditory evoked potentials using a computational model of auditory-nerve processing. The Journal of the Acoustical Society of America, 155, 1799-1812, https://doi.org/10.1121/10.0025136.
Temboury-Gutierrez, M., Märcher-Rørsted, J., Bille, M., Yde, J., Encina-Llamas, G., Hjortkjær, J., & Dau, T. (2024b). Electrocochleographic frequency-following responses as a potential marker of age-related cochlear neural degeneration. Hearing Research, 446, 109005. https://doi.org/10.1016/j.heares.2024.109005
Tremblay, K. L., Pinto, A., Fischer, M. E., Klein, B. E. K., Klein, R., Levy, S., Tweed, T. S., & Cruickshanks, K. J. (2015). Self-reported hearing difficulties among adults with normal audiograms. Ear Hearing, 36, e290-e299, https://doi.org/10.1097/AUD.0000000000000195.
Valderrama, J. T., Beach, E. F., Yeend, I., Sharma, M., Dun, B. V., & Dillon, H. (2018). Effects of lifetime noise exposure on the middle-age human auditory brainstem response, tinnitus and speech-in-noise intelligibility. Hearing Research, 365, 36-48, https://doi.org/10.1016/j.heares.2018.06.003.
Valderrama, J. T., de la Torre, A., & McAlpine, D. (2022). The hunt for hidden hearing loss in humans: From preclinical studies to effective interventions. Frontiers in Neuroscience, 16, https://doi.org/10.3389/fnins.2022.1000304.
Valero, M. D., Burton, J. A., Hauser, S. N., Hackett, T. A., Ramachandran, R., & Liberman, M. C. (2017). Noise-induced cochlear synaptopathy in rhesus monkeys (macaca mulatta). Hearing research, 353, 213-223, https://doi.org/10.1016/j.heares.2017.07.003.
Vasilkov, V., Garrett, M., Mauermann, M., & Verhulst, S. (2021). Enhancing the sensitivity of the envelope-following response for cochlear synaptopathy screening in humans: The role of stimulus envelope. Hearing Research, 400, 108132, https://doi.org/10.1016/j.heares.2020.108132.
Verhulst, S., Altoe, A., & Vasilkov, V. (2018). Computational modeling of the human auditory periphery: Auditory-nerve responses, evoked potentials and hearing loss. Hearing Research, 360, 55-75, https://doi.org/10.1016/j.heares.2017.12.018.
Viana, L. M., O’Malley, J. T., Burgess, B. J., Jones, D. D., Oliveira, C. A. C. P., Santos, F., Merchant, S. N., Liberman, L. D., & Liberman, M. C. (2015). Cochlear neuropathy in human presbycusis: Confocal analysis of hidden hearing loss in post-mortem tissue. Hearing Research, 327, 78-88, https://doi.org/10.1016/j.heares.2015.04.014.
Wojtczak, M., Beim, J. A., & Oxenham, A. J. (2017). Weak middle-ear-muscle reflex in humans with noise-induced tinnitus and normal hearing may reflect cochlear synaptopathy. eneuro, 4,ENEURO.0363- 17.2017, https://doi.org/10.1523/ENEURO.0363-17.2017.
Wu, P., Liberman, L. D., Bennett, K., de Gruttola, V., O’Malley, J. T., & Liberman, M. (2019). Primary neural degeneration in the human cochlea: Evidence for hidden hearing loss in the aging ear. Neuroscience, 407, 8-20, https://doi.org/10.1016/j.neuroscience.2018.07.053.
Wu, P., O’Malley, J. T., de Gruttola, V., & Liberman, M. C. (2020). Age-related hearing loss is dominated by damage to inner ear sensory cells, not the cellular battery that powers them. The Journal of Neuroscience, 40, 6357-6366, https://doi.org/10.1523/JNEUROSCI.0937-20.2020.
Wu, P., O’Malley, J. T., de Gruttola, V., & Liberman, M. C. (2021). Primary neural degeneration in noise-exposed human cochleas: Correlations with outer hair cell loss and word-discrimination scores. The Journal of Neuroscience, 41, 4439-4447, https://doi.org/10.1523/JNEUROSCI.3238-20.2021.
Yeend, I., Beach, E. F., Sharma, M., & Dillon, H. (2017). The effects of noise exposure and musical training on suprathreshold auditory processing and speech perception in noise. Hearing Research, 353, 224-236, https://doi.org/10.1016/j.heares.2017.07.006.
Zan, P., Presacco, A., Anderson, S., & Simon, J. Z. (2020). Exaggerated cortical representation of speech in older listeners: mutual information analysis. Journal of Neurophysiology, 124, 1152-1164, https://doi.org/10.1152/jn.00002.2020.
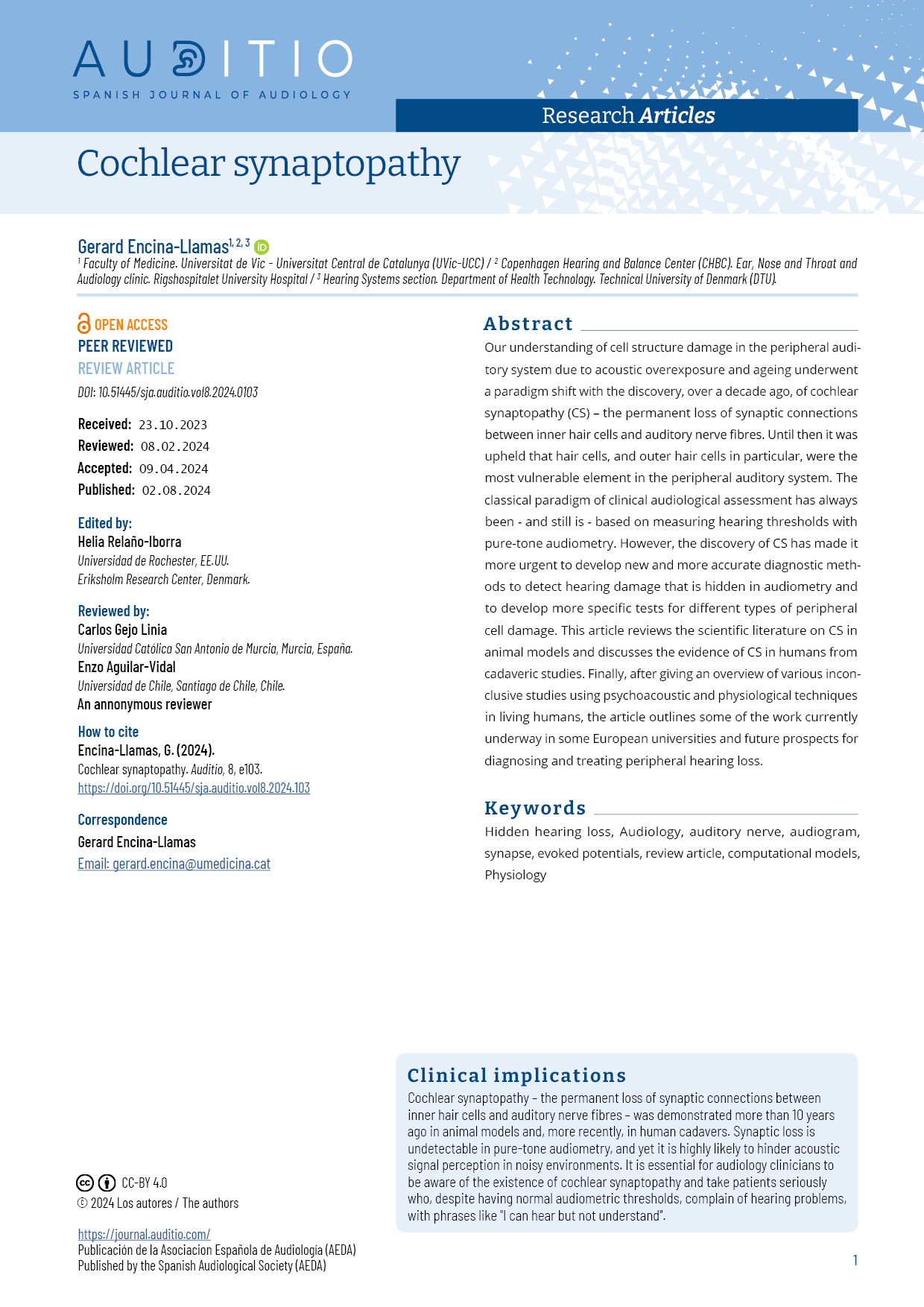
Downloads
Published
How to Cite
License
Copyright (c) 2024 Gerard Encina-Llamas

This work is licensed under a Creative Commons Attribution 4.0 International License.
Articles published after 2020
Authors retain copyright and grant the journal right of first publication with the work simultaneously licensed, unless otherwise indicated, under a Creative Commons Attribution License that allows others to share the work with an acknowledgement of the work's authorship and initial publication in this journal. The authors can choose between the following Creative Commons licenses: https://creativecommons.org/about/cclicenses/
Articles published between 2001 and 2020
The texts published in this journal in the section "AUDITIO 2001-2020" are subject - unless otherwise indicated - to a Creative Commons Attribution 3.0 Spain license. You can copy, distribute, communicate them publicly, make derivative works and commercial uses provided that you acknowledge the credits of the works (authorship, name of the journal, publishing institution) in the manner specified by the authors or by the journal. The full license can be consulted at http://creativecommons.org/licenses/by/3.0/es/deed.es.